Good vibrations for TRH
23 August 2021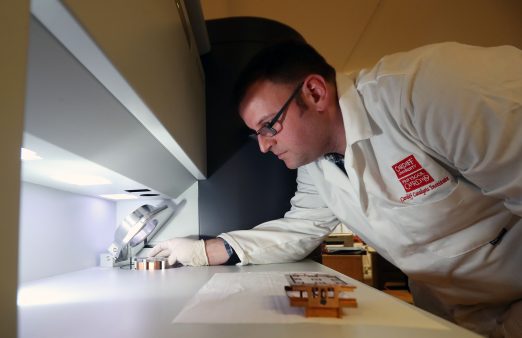
‘In scientific terms, it’s difficult to overstate the importance of the surface,’ Professor Philip Davies writes.
‘The semiconductor industry, for example, could not exist without exquisite knowledge, and control, of the surface. The chemicals industry, one of the other pillars of modern society, depends on the surface properties of nanometre-sized particles that make up the heterogeneous catalysts over which most of its products are made. Every interaction of a material with its environment occurs through the surface.
There is a good argument to be made, then, that the layer of atoms at the surface of any material is its most important. Studying the behaviour of surface atoms is crucial in almost every aspect of technology.
The difficulty is that there are so very few of them: a cubic centimetre of material contains about 1023 atoms but only about 1015 of those are at the surface. It may sound a lot, but that’s only 1 in 108 of the total.
Cardiff has been an international centre for the study of surfaces for over 50 years with expertise in many of the different experimental methods that have been developed and a particular specialisation in x-ray photoelectron spectroscopy (XPS). In fact, Cardiff currently leads the UK’s Engineering and Physical Sciences Research Council (EPSRC) National Research Facility in photoelectron spectroscopy, HarwellXPS, which provides XPS expertise for the whole country.
Our long track record of excellence in surface science, together with the fantastic facilities offered by Cardiff University’s future Translational Research Hub (TRH), have led to another ‘first’ for Cardiff: the EPSRC has awarded £1m to buy the UK’s first Photo-induced Force Microscope (“PiFM” for short).
This new technique will complement the facilities at the TRH (currently under construction on Cardiff Innovation Campus) by providing simultaneous vibrational spectroscopy and topography (in other words “shape”) of a surface with a lateral resolution of only a few nanometres.
Vibrational spectroscopy provides a crucial new insight into the chemistry of the surface by telling us about the bonds between atoms, whereas techniques such as XPS only identify the atoms themselves. Furthermore, PiFM’s ability to locate that chemistry to within a few nanometres makes PiFM an extraordinarily powerful technique for investigating the behaviour of surfaces in greater detail than has ever been possible.
On catalysts, for example, we will be able for the first time to see what reactions are occurring in the vicinity of the active nanoparticles; we can also look at the specific interactions of nanoparticulate materials on cell walls: we know that some types of nanoparticles cause cells great trouble, for the first time we will be able to study the cell wall in the immediate vicinity of the particle to find out why.
The PiFM arrangements typify the TRH interdisciplinary approach. The management team consists of researchers from Cardiff Catalysis Institute and the Institute for Compound Semiconductors, who will share the TRH building, but also scientists from the Schools of Bioscience, of Earth and Environmental Sciences, and Engineering.
In fact, scientists from nearly every other school in the Colleges of Physical Sciences and Engineering and Biomedical and Life Sciences have proposed experiments for the PiFM.
We also have great support from industry including Johnson Matthey, Delegallera, CMD and others. The instrument will be commissioned in April 2022, and once operational, it will mark a step change in our pursuit of surface science.
You can follow our progress on a dedicated web site www.pifm.uk.’
Philip Davies, Professor of Physical Chemistry, Cardiff Catalysis Institute
Figure 1. PiFM images taken during the development of a magnetic nanoparticle of hematite (Fe2O3, iron oxide).1 Image (a) shows the topography of the nanoparticle, the brighter areas showing greater height above the background. Image (b) shows a corresponding map of a vibrational signal at 910 cm-1. This wavenumber is specific to goethite (FeOOH) and so the image shows how the growth of the particle commences in the centre of the particle from the nucleation of goethite
Figure 2. PiFM images recorded from a cluster of CePO4 nanrods.2 Image (a) shows the topography of the nanoparticles, the brighter areas showing greater height above the background. Image (b) shows a corresponding map of a vibrational signal comparing the intensities of the 1409 and 1620 cm-1 peaks.